Imagine yourselves to be transported back to the end of the 19th century in 1897, at the Faculty of Medicine and Pharmacy of Lyon, France. There, you would find Ernest Ducheste, a young student in medicine performing a thesis presentation on the topic, “Contribution to the study of vital competition between microorganisms: antagonism between moulds and microbes.” He stated that “vital competition for survival occurred between fungi and bacteria, this phenomenon could be exploited for therapeutic purposes.” He used the mold Penicillium glaucum to kill Salmonella Typhi and E. coli both in vitro and in vivo. Though this was a ground-breaking idea at that time, this man and his work went unnoticed. This was until his work was validated posthumously in 1942 by Florey, Jennings, and Chain, stating that he discovered the antibiotic Patulin. His thesis was founded and he was recognized posthumously by Selman Waksman in 1945. Today, all these scientists, along with Sir Alexander Fleming, have received well-deserved Nobel prizes for discovering antibiotics. [5]
Moving on further in time, to 1929, Sir Alexander Fleming, during his research against influenza viruses, discovered that the Petri plate containing staphylococci was contaminated by the mold Penicillium notatum (now known as P. rubens). The mold had a clear zone surrounding itself where no bacteria were able to grow. He conducted experiments to unravel this unusual phenomenon. Lo and behold! Even after being diluted as much as 800 times, the bacteria were found to be killed. And thus, although by absolute chance, the first antibiotic was discovered: Penicillin! The timing of this discovery couldn’t have been better; just two decades later, these drugs were essential in fighting the many wound infections that the soldiers of World War II were falling victim to. [12]
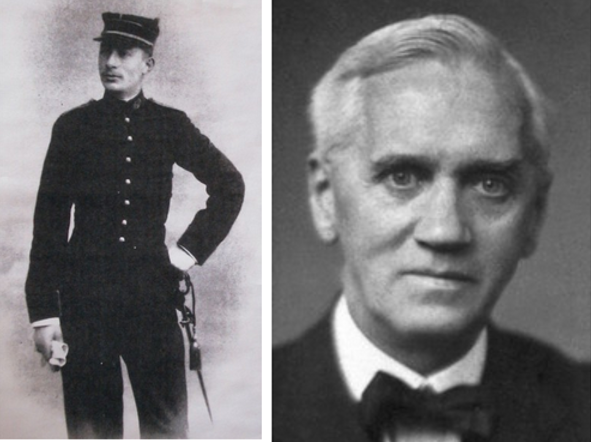
a) Ernest Duchesne (on the left), a French medical student who first discovered antibiotics in 1896.
b) Years later, Sir Alexander Fleming (on the right), who serendipitously discovered Penicillin in 1929.
Image credits: https://incubator.rockefeller.edu/geek-of-the-week-ernest-duchesne/; https://www.nobelprize.org/prizes/medicine/1945/fleming/biographical/
Since then, antibiotics have become mainstream and have saved many lives that could have been lost to fatal and complicated bacterial infections, such as tuberculosis, meningitis, sepsis, etc. Researchers have defined antibiotics as secondary metabolites or compounds produced usually by fungi or actinomycete bacteria which are used to kill any other bacteria. These compounds are produced naturally by microorganisms in response to competition for resources.
You might have heard about the scary phenomenon of emerging antibiotic resistance all over the world. It is being increasingly evident that pathogenic bacteria are gaining resistance to antibiotics. Thus, it is becoming harder to treat infectious diseases. It also leads to increased chances of mortality and economic strain due to higher costs of treatments and longer hospital duration. The obvious reason for this phenomenon is rapid bacterial cell division or growth rate, which creates numerous generations in a very short amount of time, leading to mutation and eventually enabling evolution. Other man-made reasons include their wanton use, over/under-prescription by medical professionals, not following the prescribed drug regimen regularly, not following personal hygiene etiquettes, etc. WHO observes World Antimicrobial Awareness Week (WAAW) from 18th to 24th November every year to generate public awareness to curb this problem. [2, 20]
The mechanisms used by bacteria are enzymatic deactivation (e.g.: β-lactamases that degrade penicillin drugs), drug efflux by using active transport (basically utilizing energy or ATP to throw the drug out of the cell), reducing cell entry through transporters or cell membrane, horizontal gene transfer of resistance genes, changing the target’s property, etc. This is possible either by gene transfer or mutation. Usually, these resistant bacteria are those 0.1% to 1% that survive the effects of antibiotics by chance or by using the above-mentioned mechanisms. [19]
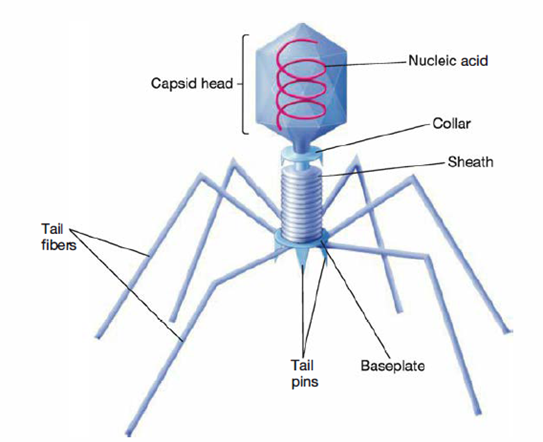
General structure of a bacteriophage, a T4 phage. This well-studied bacteriophage acts against E. coli. Derived from [13]
Today, a very viable alternative has shown itself- Bacteriophages! These are viruses that specifically infect bacteria only. It attaches only onto its particular host bacteria by lipopolysaccharides or teichoic acids or any proteins on the outermost cell wall of the bacterial cell. They inject their genome (majorly dsDNA) into the bacterial cell which then hijacks bacterial cellular machinery to replicate itself and create many new phages. After all new phage components are formed and assembled, they lyse the bacterial cell using the enzyme lysozyme or endolysin. This “reproductive” cycle of phages is the lytic cycle. In another variant called the lysogenic cycle, the genetic material integrates into the host genome and passes through a stage of dormancy, only to get reactivated by external stimuli and follow the lytic cycle. The phages that only follow the lytic cycle are called “virulent phages” whereas those that follow both cycles are called “temperate phages.” These viruses can be exploited to combat antimicrobial resistance in a process called phage therapy. This involves treating bacterial infections (normal or drug-resistant), a particular type of phage is isolated, enriched, and used. The phage should be active and not have undergone any prior degradation or modification. Phages are administered by direct subcutaneous injection near the wounds or by bandages soaked in the bacteriophage solution. In case the infection is located deeper and is not easily accessible, the area is opened by surgery and the phage solution is injected. After the work is done, the absence of their host bacteria inactivates or kills them naturally in the human host [3, 15, 18]. These days, to ensure the most efficacy, a phage cocktail consisting of many phages of different specificities is supplied [9].
The following is a list of pros and cons of the therapeutic use of phages [7, 8, 14]:
Pros:
1. Very narrow activity spectrum, which avoids the killing of resident microflora of the host along with the pathogen; this clearance of the resident microflora could lead to superinfections of pathogens like Clostridium difficle, which causes chronic and fatal diarrhoea.
2. Phage-infected bacteria lose their viability and cannot multiply.
3. Bacteriophages have been detected to be ubiquitous in almost all environments on Earth and can be easily found.
4. Minimal harm and nontoxic to human host cells since only the bacterial cells are targeted.
5. Single administration is sufficient for treatment. Phages multiply rapidly upon infecting the target bacteria; this leads to “auto-dosing,” which means that phages adjust their density according to the bacterial population density.
6. Resistance is not induced easily because of phage specificity.
7. Can be modified by genetic engineering and biotechnology.
8. Versatile mode of administration.
9. Has the capacity to remove biofilms, which are hardy films of bacterial cells and exopolysaccharides (like mucus) that make them very difficult to treat by antibiotics since penetration and access are difficult.
10. Sustainable and cheap as they can be easily handled and stored.
11. Works against both resistant and normal strains.
Cons:
1. Human immune response can be elicited by the presence of bacteriophages.
2. Identifying bacteriophages that have therapeutic potential and do not cause any adverse side effects can be difficult as the phage genome could have any toxic genes coding for virulence factors.
3. Even though the chance is low, bacteriophages could also contribute to antimicrobial resistance.
4. Phage therapy may not work against bacteria that have defence mechanisms against viruses, such as certain enzymes (e.g.: restriction endonucleases) and proteins (e.g.: CRISPR).
5. Questions related to the functionality and durability of pharmaceutical preparations, whether they will maintain the active phages.
6. Not enough therapeutic phages might be available for every bacterium (normal or multidrug-resistant).
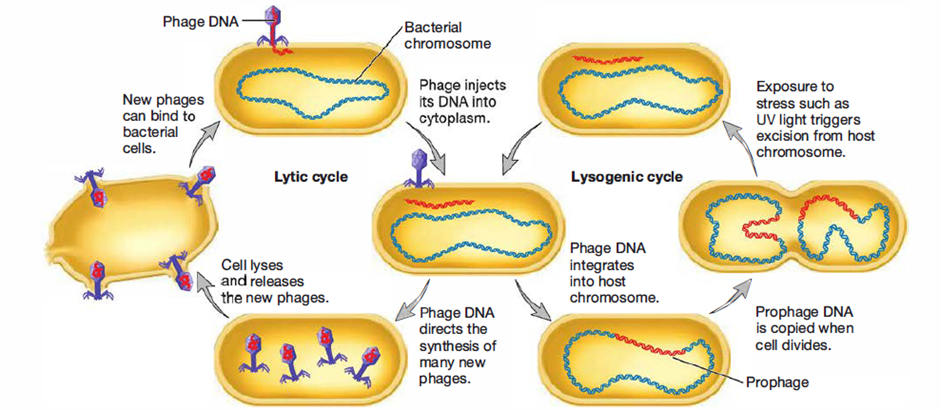
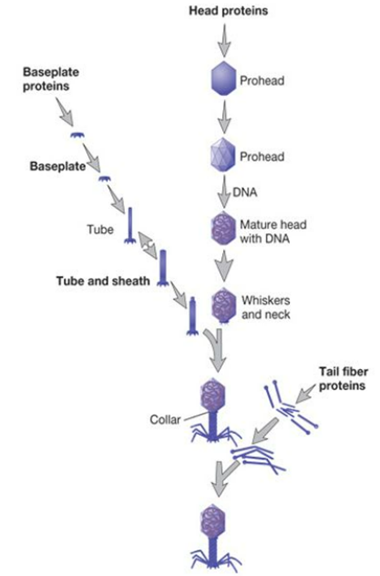
a) Overview of both lytic and lysogenic cycles
b) General structure of a bacteriophage.
Both are from [13]
Phage therapy has an interesting history intermixed with the politics around the blank period leading to WW2. Phages were first discovered by the English scientist William Twort in 1915. Their potential as a deterrent to the bacterial pathogen of dysentery by causing their lysis was realized by Felix d’Herelle. He described this phenomenon as an “invisible microbe antagonistic to the dysentery bacillus.” He was pretty convinced that this was because of a microorganism other than bacteria, i.e., a virus, and named this organism a “bacteriophage” based on his observations. Scientists like Bordet and John Northrop opposed his viewpoint and claimed that lysis was caused by an enzyme instead. Eventually, in 1939, after the invention of the electron microscope in Hitler’s pre-war Germany, his claims were validated. But this was not shared with the USA because of the tensions and the impending WW2. Eventually, the images of the phages were released by the French press. Before WW2, from the 1920s, the Soviet Union showed an unparalleled interest in bacteriophage research because of the mystery surrounding it. Till the 1940s, American scientists released analytical reports disproving phage therapy because of their personal biases, clinical inadequacies in standardization, and the ongoing testing of other therapeutic molecules at that time. Hence, this was considered suspicious and till that time, antibiotics had proven to be more feasible than culturing or maintaining phages. Even after all this, no one could dampen the spirits of the Soviet Union scientists who went ahead to carry out phage therapy clinical trials in the Donbass region in 1929 and 1935 against pathogens of dysentery, scarlet fever, and typhoid. These first trials turned out to be successful. D’Herelle travelled extensively to countries such as India, Brazil, Egypt, etc. to carry out clinical trials against these pathogens and pathogens of cholera and plague. He had produced these bacteriophage solutions in his lab in Paris, France, which were advertised then by the French company L'Oréal as “Bacté-coli-phage,” “Bacté-rhino-phage,” “Bacté-intesti-phage,” “Bacté-pyo-phage,” and “Bacté-staphy-phage.” [4, 11, 16, 17]
During Stalin’s Reign of Terror in the 1930s, the lives of several pioneering phage therapy scientists of that time were claimed. This included Giorgi Eliava who in 1923, along with Felix d’Herelle, founded the institute that is currently providing the best phage therapy at Eliava Phage Therapy Center from the Eliava Institute of Bacteriophage, Microbiology, and Virology in Tbilisi, Georgia (EIBMV). Learn more about these two here (https://eptc.ge/about-eptc/ & https://pha.ge/?lang=en). Eventually, the USSR and sometimes the Germans resorted to treating bacterial infections using phage therapy because only the other Allied powers (France, Great Britain, the United States) except USSR had access to antibiotics. Other Eastern bloc countries which were satellite states to the USSR at that time used the same treatment. These countries surprisingly report that in the 1940s, American and European pharmaceutical companies were extensively producing and developing bacteriophages for treatment [3, 15]. After WW2 ended, research from the USSR was viewed under suspicion by the Allies because of certain pseudoscientific claims and the rise of communism. This made the prospects of phage therapy in the West bleaker. Thus, antibiotics eventually became political and thanks to the Cold War, become more popular in the West and in countries that allied with the USA. Also, the rise of ‘Big Pharma’ further led to the downfall of phage therapy, and it stopped in the early 1970s. Antibiotics were considered ‘wonder dugs’ that had a lot of advantages on their side: better efficacy, easier administration, stability, easier to make, etc. [4, 11, 17]
The history of phage therapy in Brazil, South America, is also notable. Although this bit of history seems to contribute majorly to the legacy of phage therapy, it has been scarcely cited; only around three times. The Brazilian pioneer scientist is Dr. José da Costa Cruz from the Oswaldo Cruz Institute, Rio de Janeiro, Brazil. The research and the clinical trials he conducted lasted from 1921–24 and preceded those of D’Herelle. Dr. José successfully established a proper treatment for staphylococci and bacterial dysentery. Unfortunately, the same political events happened in Brazil, and antibiotics replaced phages. [1]
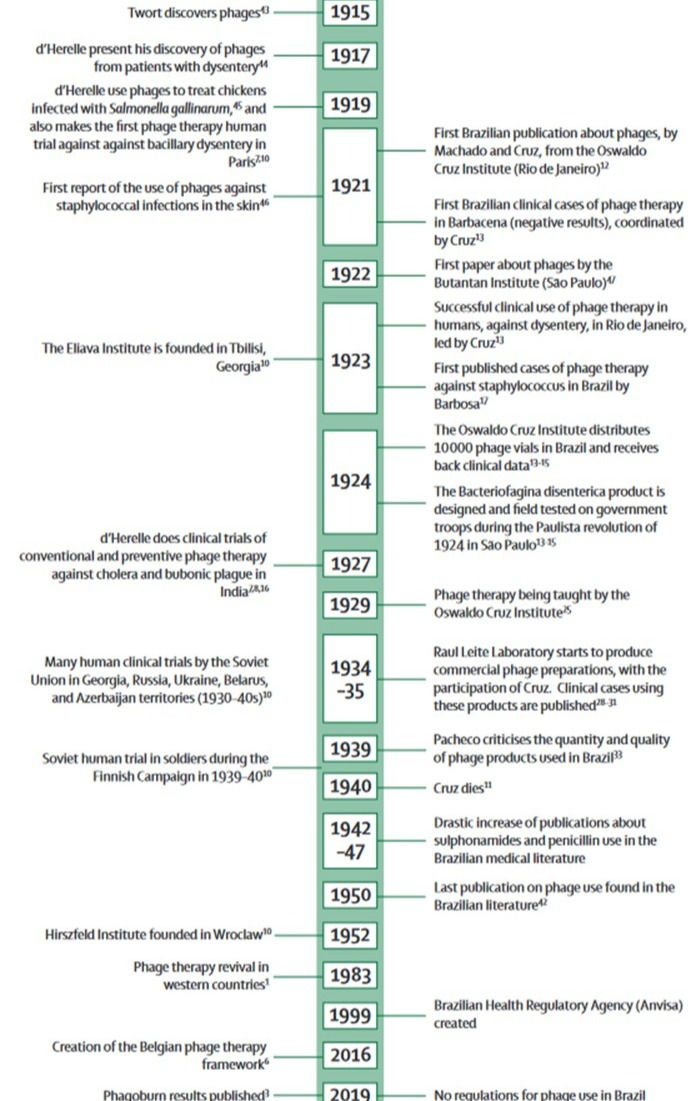
History of phage therapy throughout the world and Brazil. Derived from [18]
The wonderfulness of antibiotics seems to have fizzled out in a decade as reports emerged of phage therapy being recommended for antibiotic resistance in the 1980s. Today, phage therapy is mainstream in Georgia (EIBMV), Russia (many companies and medical centres) and Poland (Hirszfeld Institute of Immunology and Experimental Therapy / HIIET), and western countries such as the USA and Europe are still clinically testing it. Belgium has aimed to streamline the pharmaceutical process through the Belgian phage therapy network. [1, 10, 13]
The future for this therapy seems bright as scientists all over the world are realizing the demerits of antibiotics. It seems better to tweak nature’s phenomenon of “predator eats prey” and use it to our advantage. In India, this therapy is picking up pace and few labs and diagnostic centres have started working to make it more accessible to the Indian Public and build awareness. Some of them are Dr. Dang’s lab (https://www.drdangslab.com/ServiceBacteriophageSenstivityTesting.aspx), Vitalis Phage therapy (https://www.vitalisphagetherapy.com/) and Dr. Ramesh N.’s lab at VIT (https://sites.google.com/view/rameshnachimuthulab). I sincerely hope that their efforts bear fruit!
References
[1] Almeida, Gabriel Magno de Freitas, and Lotta-Riina Sundberg. 2020. “The Forgotten Tale of Brazilian Phage Therapy.” The Lancet Infectious Diseases 20 (5): e90–101. https://doi.org/10.1016/S1473-3099(20)30060-8.
[2] Australian Government. 2017. “What Causes AMR? | Antimicrobial Resistance.” Antimicrobial Resistance. Australian Government. July 25, 2017. https://www.amr.gov.au/about-amr/what-causes-amr Updated on 31st October 2017. Accessed on 1st Dec 2021
[3] Brives, Charlotte, and Jessica Pourraz. 2020. “Phage Therapy as a Potential Solution in the Fight against AMR: Obstacles and Possible Futures.” Palgrave Communications 6 (1): 1–11. https://doi.org/10.1057/s41599-020-0478-4.
[4] Clokie, Martha R.J., Andrew D. Millard, Andrey V. Letarov, and Shaun Heaphy. 2011. “Phages in Nature.” Bacteriophage 1 (1): 31–45. https://doi.org/10.4161/bact.1.1.14942.
[5] Duckett, Serge. 1999. “Ernest Duchesne and the Concept of Fungal Antibiotic Therapy.” The Lancet 354 (9195): 2068–71. https://doi.org/10.1016/s0140-6736(99)03162-1.
[6] Gordillo Altamirano, Fernando L., and Jeremy J. Barr. 2019. “Phage Therapy in the Postantibiotic Era.” Clinical Microbiology Reviews 32 (2). https://doi.org/10.1128/cmr.00066-18.
[7] Iftikhar, Noreen. 2019. “Phage Therapy: How It Works, Pros and Cons, Availability, and More.” Healthline. 2019. https://www.healthline.com/health/phage-therapy.
[8] Loc-Carrillo, Catherine, and Stephen T. Abedon. 2011. “Pros and Cons of Phage Therapy.” Bacteriophage 1 (2): 111–14. https://doi.org/10.4161/bact.1.2.14590.
[9] McCallin, Shawna, Shafiqul Alam Sarker, Caroline Barretto, Shamima Sultana, Bernard Berger, Sayeda Huq, Lutz Krause, et al. 2013. “Safety Analysis of a Russian Phage Cocktail: From MetaGenomic Analysis to Oral Application in Healthy Human Subjects.” Virology 443 (2): 187–96. https://doi.org/10.1016/j.virol.2013.05.022.
[10] Międzybrodzki, Ryszard, Naomi Hoyle, Fikria Zhvaniya, Marzanna Łusiak-Szelachowska, Beata Weber-Dąbrowska, Małgorzata Łobocka, Jan Borysowski, et al. 2018. “Current Updates from the Long-Standing Phage Research Centers in Georgia, Poland, and Russia.” Bacteriophages, 1–31. https://doi.org/10.1007/978-3-319-40598-8_31-1.
[11] Myelnikov, Dmitriy. 2018. “An Alternative Cure: The Adoption and Survival of Bacteriophage Therapy in the USSR, 1922–1955.” Journal of the History of Medicine and Allied Sciences 73 (4): 385–411. https://doi.org/10.1093/jhmas/jry024.
[12] Nobel Prize. 2019. “The Nobel Prize in Physiology or Medicine 1945.” NobelPrize.org. 2019. https://www.nobelprize.org/prizes/medicine/1945/fleming/biographical/.
[13] Pirnay, Jean-Paul, Gilbert Verbeken, Pieter-Jan Ceyssens, Isabelle Huys, Daniel De Vos, Charlotte Ameloot, and Alan Fauconnier. 2018. “The Magistral Phage.” Viruses 10 (2): 64. https://doi.org/10.3390/v10020064.
[14] Principi, Nicola, Ettore Silvestri, and Susanna Esposito. 2019. “Advantages and Limitations of Bacteriophages for the Treatment of Bacterial Infections.” Frontiers in Pharmacology 10 (513): 1–9. https://doi.org/10.3389/fphar.2019.00513.
[15] Reardon, Sara. 2014. “Phage Therapy Gets Revitalized.” Nature, June 2014. https://doi.org/10.1038/510015a.
[16] Sulakvelidze, A., Z. Alavidze, and J. G. Morris. 2001. “Bacteriophage Therapy.” Antimicrobial Agents and Chemotherapy 45 (3): 649–59. https://doi.org/10.1128/aac.45.3.649-659.2001.
[17] Summers, William C. 2012. “The Strange History of Phage Therapy.” Bacteriophage 2 (2): 130–33. https://doi.org/10.4161/bact.20757.
[18] Willey, Joanne M, Linda Sherwood, and Chris Woolverton. 2013. Prescott’s Microbiology:9th Revised Edition. 9th ed. London: Mcgraw Hill Higher Education.
[19] Wilson, Brenda A, Abigail A Salyers, Dixie D Whitt, and Malcolm E Winkler. 2011. “How Bacteria Become Resistant to Antibiotics.” In Bacterial Pathogenesis: A Molecular Approach, 3rd ed., 347–70. Washington, Dc: Asm Press.
[20] World Health Organization (WHO). 2020. “Antibiotic Resistance.” World Health Organization. July 31, 2020. https://www.who.int/news-room/fact-sheets/detail/antibiotic-resistance.
Comments